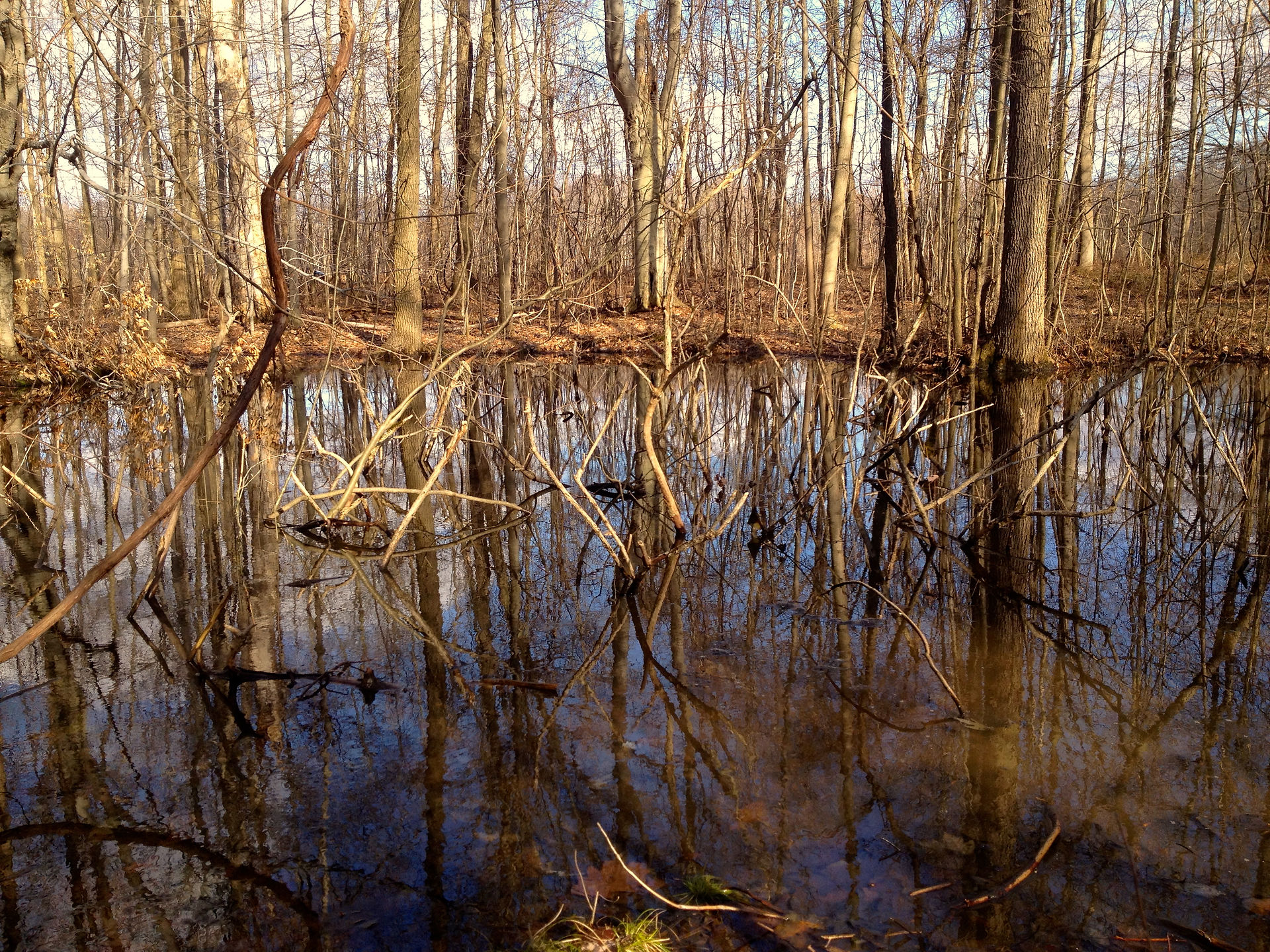
-Purdue University-
Devin K. Jones

Understanding human effects on freshwater systems
Natural ecosystems are increasingly threatened by numerous abiotic and biotic factors, including those that arise from anthropogenic sources. For example, pesticide use to control pest species and disease vectors has dramatically increased since the 1940s, and has led to the ubiquitous contamination of natural systems. It is estimated < 1% of applied pesticides reaches targeted species; the remaining 99% can enter natural systems through multiple pathways, including improper use and disposal, run-off, direct overspray, and atmospheric drift and deposition. Researchers aim to investigate and understand both lethal and sublethal effects on organisms inhabiting these contaminated ecosystems to mitigate negative effects on wildlife and humans alike.
​
​
Laboratory toxicity tests
The lethal and sublethal effects of pesticides have traditionally been studied using short-term (4 day), single-species laboratory toxicity tests. Toxicity tests are useful for investigating the toxic effects of numerous pesticides over a short period of time.
​
As an undergraduate research assistant and REU, I employed a variety of toxicity tests to investigate toxic effects of two commonly applied pesticides. First, I investigated the direct lethal effects of Roundup (glyphosate) on 13 amphibian species, including 9 anurans and 4 salamanders (Relyea and Jones 2009). Although laboratory toxicity tests investigate toxic effects of active ingredients, few test the effects of formulated products, which include the active ingredient and other inert chemicals (e.g., surfactants). We observed similar levels of sensitivity among our anuran and salamander species, and found that Roundup was more toxic to frog species as compared to salamanders. The inclusion Cascades frogs, western toads, and the 4 salamander species in our study filled important gaps in the literature, as well as added to the growing database available to researchers to provide accurate risk assessments.
​
Second, I conducted toxicity tests to investigate the sensitivity of amphibian species from three families to the insecticide endosulfan (Jones et al. 2009, Hammond et al. 2012). Following a 4-day exposure, all exposed individuals were moved to clean water and were observed for latent effects of endosulfan exposure. We found that the initial 4-day endosulfan exposure caused increased mortality in tadpoles beyond the standard 4-day testing period. Moreover, we found phylogenetic signals in amphibian sensitivity to endosulfan and the appearance of lag effects. Our results indicate that standard 4-day toxicity tests may not properly predict lethal effects on non-target species, and added to the body of literature used to support the decision to phase-out endosulfan use in the United States.
​
​
​
​
Ecotoxicology​
Although toxicity tests are important to assess initial toxicity of anthropogenic chemicals, they fail to include many of the complexities found within natural systems. Outdoor mesocosm experiments that mimic natural aquatic systems have revealed numerous interactions among natural stressors (e.g., predation, competition) and pesticides that are otherwise absent in simplified laboratory experiments.
Pesticide application varies with active ingredient, which influences the recommended application concentration and the timing and frequency of exposures. Using outdoor mesocosms, I investigated the direct and indirect effects of Roundup application timing, frequency, and concentration on semi-natural vernal pool communities (Jones et al. 2010). Amphibian mortality was higher when Roundup was applied earlier in the experiment than later in the experiment. Moreover, a single, large application had larger negative effects on tadpole survival and growth than multiple, small applications of the same total amount. Our results show that tadpoles become more tolerant over development, and acclimate to toxic concentrations of the herbicide following multiple, small applications.
​
We then manipulated the density of our semi-natural pond communities to investigate the potential synergistic effects of competitive stress and Roundup (Jones et al. 2011). Under high competition, sublethal concentrations of Roundup became lethal to American bullfrogs (Lithobates catesbeianus). It is of utmost important to conduct future experiments under more natural conditions before extrapolating results of laboratory experiments, as many of the effects and interactions change as the complexity of experimental conditions increases.
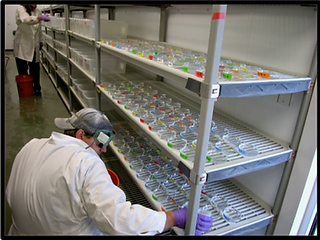
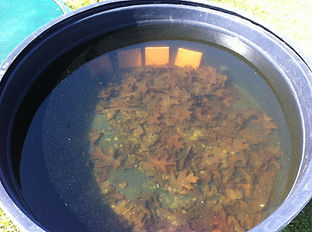

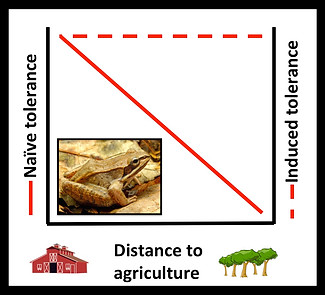
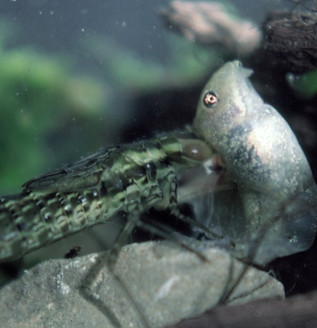
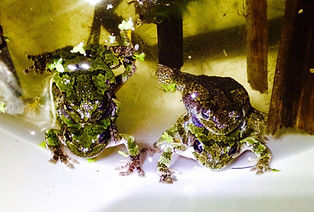
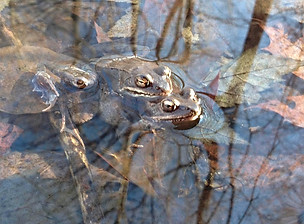
Evotoxicology
The evolution of pesticide tolerance causes over $1.5 billion in losses annually in the United States, and has been documented in more than 500 species. Historically, evolutionary responses to pesticides in exposed populations has been thought of as a genetic response following the selection on the constitutive expression of a trait that confers increased tolerance. Surprisingly, Increased tolerance to pesticides has not only been reported in populations of pest species, but non-target species as well (Cothran et al. 2013, Hua et al. 2013, Bendis and Relyea 2014, Hua et al. 2015). Populations of non-target species nearest agriculture exhibit the highest level of constitutive (naïve) tolerance, whereas populations farthest from agriculture are extremely sensitive to agrochemicals (Hua et al. 2013, Hua et al. 2015).
Recent research, however, has shown that some species are able to respond plastically, within one generation, to increase pesticide tolerance (Hua et al. 2013). Moreover, only populations far from agriculture are able to induce increased pesticide tolerance following sublethal exposures early in development (Hua et al. 2013, Hua et al. 2015). Yet, little is known why populations far from agriculture and agrochemical exposure are able to induce adaptive responses to a stressor rarely encountered. Furthermore, evolutionary responses to agrochemicals (i.e., constitutive vs. inducible tolerance) might have influence plastic responses to other stressors.
I asked if the ability to induce an adaptive response to sublethal pesticide concentrations was the result of a "general-stressor response" following exposure to any anthropogenic or natural stressors early in life (Jones et al. Under review). I exposed wood frog tadpoles to sublethal anthropogenic (two concentrations of the commonly applied insecticide carbaryl, two concentrations of road salt (sodium chloride, NaCl) and natural (Anax junius [larval dragonfly] cues) stressors for approximately 4 days and tested their subsequent tolerance to a lethal carbaryl dose. Not surprisingly, sublethal exposure to carbaryl induced increased tolerance to the lethal carbaryl concentration. However, early exposure to dragonfly larvae cues induced increased tolerance to the lethal carbaryl concentrations. Our results suggest that the ability to induce pesticide tolerance likely arose through anti-predator responses and that predator- and pesticide-induced responses have a shared evolutionary pathway. Given that anti-predator responses are widespread in prey species, it might be that a wide diversity of animal taxa possess inducible pesticide tolerance, thereby buffering populations from deleterious effects of agrochemicals.
​
Given the potentially shared evolutionary pathway between inducible responses to predators and pesticides, I (Jones et al. 2021) then asked if plastic responses to natural stressors (e.g., competition and predation) have been altered by the evolutionary responses to pesticides (e.g., constitutive vs. inducible tolerance).
​
Phenotypic plasticity and life-history tradeoffs
Phenotypic plasticity-the ability of a single genotype to produce multiple phenotypes in response to environmental conditions-enables species to persist in heterogeneous environments. Plastic responses to environmental stressors occur rapidly (i.e., within one generation), enabling organisms to respond to their immediate environment. Although plastic responses in contaminated systems may benefit individuals, the maintenance and production of increased pesticide tolerance may incur a fitness cost. Moreover, the factors that influence the induction and retention remain unknown.
I investigated the ability of gray treefrog (Hyla versicolor) tadpoles to induce increased tolerance to pesticides, and the potential costs at metamorphosis associated with inducing a plastic response (Jones and Relyea 2015). Although gray treefrog tadpoles induced tolerance following sublethal exposure to carbaryl, the mass at metamorphosis, snout-vent length, and time to metamorphosis of gray treefrogs was not influenced by early sublethal exposures.
​
I then asked if the timing and frequency of pesticide exposure influences the induction and retention of pesticide tolerance (Jones et al. Under review). Plasticity theory describes important developmental windows that are sensitive to environmental stressors in species with complex life cycles. Combining plasticity theory and tools from ecotoxicology, I exposed juvenile wood frogs (Lithobates sylvaticus) to sublethal concentrations of the insecticide carbaryl at various developmental windows, and found 1) induced tolerance to pesticides was limited to developmental windows early and midway through tadpole development, 2) naïve tolerance of wood frog tadpoles increased with development, 3) induced tolerance was retained for a period of 1-6 days, and 4) induced tolerance to pesticides was only retained throughout development when individuals were exposed to a "press" treatment (e.g., exposed at each developmental window [4 times]). Our results show that, like responses to natural stressors (e.g., predation), the induction and retention of increased tolerance to pesticides is limited to early sensitive developmental windows and dependent upon consistent, reliable cues.